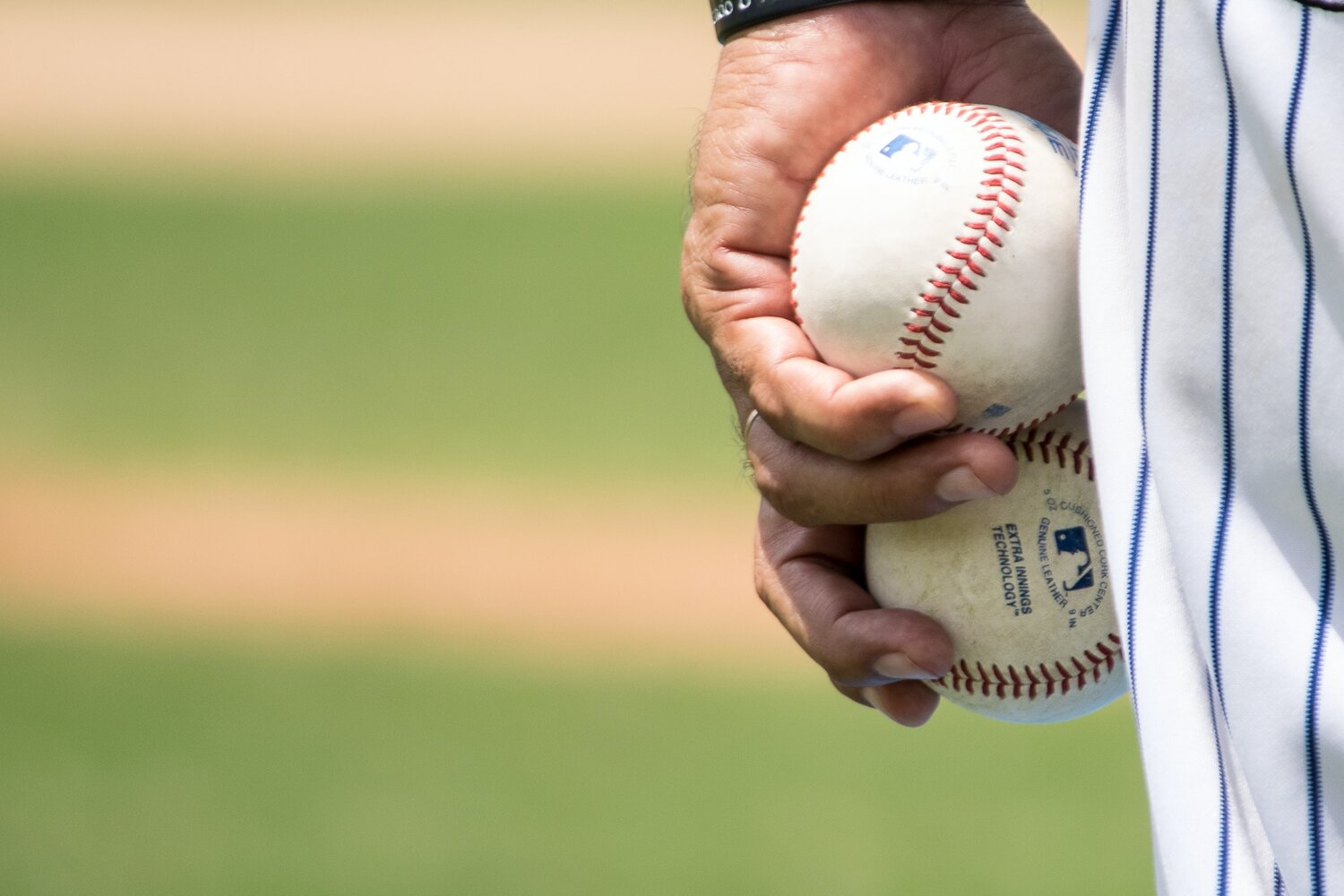
A Thought Experiment
In a previous article we described a model of the pitching mechanics by which Steve Dalkowski could plausibly have propelled a fastball to 110 mph or faster. In this article we address the related question of the physical characteristics required for Dalkowski to pitch at speeds of 110 mph or faster. Specifically, we attempt to answer how Dalko’s physical form would have needed to be optimized for him to pitch this fast.
To answer this question, we engage in a thought experiment. When the gods on Mount Olympus wanted something built for their enjoyment, they would call on the artisan god Hephaestus. No invention was too difficult for Hephaestus. If the gods could request it, he could build it. Thus Mount Olympus was populated with a wonderful array of toys, built by Hephaestus, for the amusement of the gods.
Suppose one day that Zeus, looking down from Mount Olympus and taking a fancy to baseball, asked Hephaestus to build him the perfect human pitcher. What would such a pitcher look like? As a human, the pitcher could not be a machine, like a catapult. Moreover, as an actual human, the pitcher would need to be not too extraordinary. For instance, he would lack freakish size or strength, such as the ability to benchpress a railway tank car (like Mr. Incredible in the Pixar animated superhero film). What, then, about this human would make him the perfect pitcher? If Zeus were to descend with this pitcher from Mount Olympus, how could we know that this was in fact the perfect pitcher?
Our goals in fleshing out this thought experiment are the following:
1) to dispel some of the misinformation surrounding Dalkowski’s pitching speed;
2) to consolidate various/disparate reports about what Dalkowski truly did when he pitched;
3) to create a narrative about what would have had to occur on the mound for anyone to throw a baseball between 110 and 120 mph;
4) to put to work today’s best science and biomechanical knowledge to explain Dalkowski’s speed;
5) to substantiate this narrative with solid numbers, as they exist; and
6) to draw lessons from this thought experiment for actual pitching based on the degree to which actual pitchers approximate the perfect pitcher.
Here is what we are not doing:
1) saying that Steve Dalkowski actually or necessarily did this, i.e., that he matched up perfectly with our “perfect pitcher”;
2) creating a model for pitchers to follow or emulate;
3) proving that the arm could hold up under the stresses of throwing in this “rare air.” Current science regards the arm as unable to endure the stress of throwing a baseball much faster than it is currently being thrown, let alone at 110 to 120 mph. Thus, based on the current science and knowledge of pitching, throwing a baseball this hard is viewed with extreme skepticism. Injury, even severe injury, is widely considered as the likely result of throwing a baseball this hard; and
4) concluding with finality that 110 to 120 mph can be achieved.
In fleshing out our thought experiment, we make the following assumptions:
1) that the pitcher is biomechanically perfect, no flaws;
2) that in attempting to characterize optimal pitching mechanics, it’s possible to arrange the motions and forces of the relevant body parts in an optimal sequence (thereby “cracking the whip”);
3) that a perfect pitcher could potentially “crack the whip” more effectively than any presentday pitchers;
4) that it is reasonable to build on the currently highest velocity recorded for a Major League pitcher (Aroldis Chapman’s 105 mph) in determining whether 110 to 120 mph might be realized in an absolutely perfect pitching environment (both anatomically and biomechanically).
The Context
Pitching a baseball is a highly complex motor skill. Precisely sequenced muscle recruitment patterns are required to propel a 5-ounce baseball 60’ 6” toward a target, where pitch location, pitch movement, and pitch velocity form a triad of measures of success. Generating ground force translation (linear movement toward the target) and axial skeletal rotation to develop torque, provides a slingshot effect through the throwing arm. The delivery of the baseball is a powerful anaerobic event lasting under 3.5 seconds for the professional pitcher.
Proper pitching mechanics use the motions and forces of all relevant body parts in an optimal sequence to generate what is commonly referred to as “cracking the whip.” This kinetic chain is supposed to create a perfect flow of energy coming up through the body: hips to shoulders to elbow/forearm and, ultimately, to the hand and ball. The perfect pitcher attempts to be at the right place at the right time in the pitching motion. The sequence would have to be perfect for a pitcher to experience the maximum of his potential, as anything out of sequence or out of proportion would lead to inefficiency and the potential for injury.
In giving life to our thought experiment, we will evaluate six major areas where a pitcher could potentially raise his velocity. We are going to focus on (1) the physical form (especially the musculoskeletal system), (2) timing and tempo, (3) relationship of the front hip and throwing shoulder angles, (4) stride length and leg lift, (5) arm speed, and (6) release point. The thought experiment thus attempts to identify all of the individual components in the kinetic chain that could potentially raise the velocity of a pitcher’s fastball. Finally, we assess the cumulative effect of these components and how together they could plausibly move a pitcher into the 110 to 120 mph range.
(1) Physical Form of the Perfect Pitcher
In characterizing the physical form needed for a pitcher to throw a baseball as hard as possible, we are looking for the potential advantages offered by genetics, general strength, conditioning, musculoskeletal system, recovery, and nutrition. Each component could incrementally improve a pitcher’s overall effectiveness and speed. Of these, it seems the musculoskeletal system provides the most obvious opportunity to look for an advantage: bone size (especially length), muscles to support the movement of the bones, and the connective tissue (tendons and ligaments) to hold bones and muscle together.
Studies detailing the precise connection between musculoskeletal system and pitching dynamics do not yet exist, but it makes sense to think that if the humerus, radius, and ulna were bigger, longer, and stronger, and worked in concert with muscles that had been trained or developed to be larger and stronger to handle the increased load, then the connective tissue (ligaments and tendons) would have to be exceptionally strong to prevent the arm from coming apart.
Accordingly, the potential advantages that an ideal musculoskeletal system could confer on pitching would depend crucially on enhanced strength of this connective tissue. The challenge raised by this possibility, however, is that there is currently no known way in which to significantly train, develop, improve, or strengthen ligaments and tendons (in contrast to muscles, which grow and adapt much more readily). Platelet-rich plasma (PRP) and stem cell injections, for instance, show promise of healing stressed ligaments and tendons, but at best restore these tissues to health, not make them stronger than they once were.
It thus becomes an interesting question how much variability there is in the connective tissue of humans and how strong this connective tissue could be in a perfect human pitcher. Did Dalko’s arm have the best ligaments and tendons of any professional pitcher? Perhaps, but we have no way of confirming this. That said, our perfect pitcher, especially to the degree that he was durable, would need such connective tissue.
The closer the body’s kinetic chain gets to the object being accelerated (i.e., the ball), the more likely that the advantages of longer bones and stronger muscles would need to be realized. The shoulder (proximal) would need to be strong and stable to handle the energy going down the arm— specifically shoulder to elbow, elbow to wrist, wrist to fingers, and fingers to ball. It is in principle possible for a pitcher to display all of the advantages so noted: longer bones in each segment from the shoulder to the fingers, larger and stronger muscles to support the longer bones, and abnormally (or should we say “supranormally”) stronger/denser connective tissue by which the arm delivers its motion. With all these pieces falling properly into place, there is certainly potential of increased velocity, though the timing of the segments then becomes critical (see next point).
In this kinetic chain, longer fingers are least likely to be over-stressed when making an additional contribution to velocity, followed by a more substantial wrist, forearm, elbow, and shoulder—the latter two receiving the most stress. The picture so sketched is consistent with elbow and shoulders sustaining the greatest amount of wear and tear, as baseball pitching injuries (and surgeries) readily confirm. This picture of an optimal musculoskeletal system for pitching would be further enhanced with abnormal/supranormal connective tissue—in other words, thicker ligaments, stronger tendons, and the ability to repair, heal, and recover these more quickly after throwing. These advantages could be additionally enhanced with excellent nutrition and proper rest, thus further speeding overall recovery.
Besides bigger bones and stronger connective tissue, faster fast twitch muscles could also contribute to elevated velocity. Because fast twitch fibers use anaerobic metabolism to create fuel, they are better at generating short bursts of strength and speed than slow twitch muscles. However, they do fatigue more quickly. Fast twitch fibers generally produce the same amount of force per contraction as slow twitch muscles, but they are able to fire more rapidly. Having more fast twitch fibers could be an asset to a pitcher since he needs to generate force quickly. Such a neuromuscular system would fire faster, translating to a faster pitching motion.
Finally, if a pitcher were double jointed, possessing hypermobility in his joints with the flexibility and ability to use it to deliver the ball, he could have an additional advantage for elevating velocity. Increased external shoulder rotation could lead to greater arm and ball velocities, as the pitcher would have a greater range of motion for his shoulder torque to accelerate the arm.
All of the musculoskeletal advantages just described would, in principle, enable a pitcher to throw a baseball harder. That said, before we move to the next point, a caveat is in order: sheer size must work effectively with other features of the physical form to achieve optimal pitching speeds—sheer size by itself is meaningless. Aroldis Chapman, for instance, is 6’ 4”, which is on the larger side as contemporary pitchers go.[14] But Nolan Ryan, whose unofficial fastest pitch exceeded 108 mph and thus was faster than Chapman’s 105 mph, was only 6’ 2”. Randy Johnson threw fast, exceeding 100 mph, but not in that rare air. He was 6’ 10”. The tallest MLB player ever was also a pitcher, Jon Rauch, who despite measuring 6’ 11” pitched an unexceptional low 90s fastball.[15]
Dalko was, by contrast, a mere 5’ 11”, so the advantages described here would need to be applied to his frame. Would he have approximated the perfect pitcher from Mount Olympus more closely if he had been taller? Or perhaps he was the perfect pitcher in the 6’ and under category. The factors in play here are varied and complicated.
(2) Timing and Tempo
Our second area where a pitcher could potentially raise his velocity is timing and tempo. We define timing as the order of events in throwing a baseball, and tempo as the duration in which these events are realized. Our concern in this section will be with the optimal timing of elements in the kinetic chain while also increasing the tempo of delivering the baseball.
Current biomechanical studies and testing conducted and published by Dr. Glenn Fleisig and his colleagues at the American Sports Medicine Institute (ASMI) have produced optimal time frames for each portion of the kinetic chain based on a database that records the timing and tempo of hundreds of professional pitchers. These studies indicate that there is no one right way for frontline professional pitchers to execute their pitching motion, though individual pitching motions can be performed in more, or less, efficient ways.[1-5]
Some ranges of numerical values for timing seem to hold quite generally. For example, from the first forward movement toward home plate to the front foot landing seems to occur optimally at between 0.95 and 1.05 seconds. Ball release occurs at between 1.25 and 1.35 seconds. While there are a few professional pitchers who fall outside these ranges, their numbers occur in the tails of the bell curve. However, we do know from studies conducted by Fleisig, Urban, and others at ASMI that quicker execution will produce greater ball velocity and greater joint kinetics.[6]
In the last section we noted that a pitcher’s physical advantages could significantly raise his velocity only if his kinetic chain were properly (and better yet perfectly) timed. If that cannot be done, the risk of injury, immediate and severe, would be greatly elevated and any physical advantages would be negated. On the other hand, if a pitcher possessed all the physical advantages noted earlier and could then efficiently/perfectly translate that energy from the lower half of the body, to the hips, to the shoulders, to the elbow, to the forearm, to the wrist/fingers, and finally to the baseball, while increasing the tempo of each increased handoff of energy, the sky could be the limit. A pitcher’s velocity would surely increase significantly, not simply because each individual piece of the motion added a small incremental improvement but rather because the pieces together, with timing and tempo optimized, would be perfectly aligned, yielding a collective energy that vastly increased pitching velocity.
(3) Dissociation of the Pelvis and Upper Trunk
Torque is the next point in our examination of where and how pitching velocity might increase. As the pitcher moves toward home plate, which leads to setting the lead foot on the ground, the pelvis must begin to open toward home plate (when the pelvis is fully open, the belly button is facing home plate). As the pelvis begins to open, the upper trunk remains loaded facing third base (right-handed pitcher) or first base (left-handed pitcher). The upper trunk has not yet begun to rotate. The longer the shoulder can be prevented from prematurely opening, the greater the torque and thus the rotational energy between the lower and upper trunk.
According to Dr. Tom House, whose scientific studies of pitching have been ground breaking, this dissociation of pelvis and upper trunk may produce up to 80 percent of the energy applied to the baseball, which will then translate into increased pitching velocity.[9-12] Various biomechanical studies indicate that the dissociation between pelvis and upper trunk should be between 40 and 60 degrees.[2,5,7]
A few professional pitchers exceed these benchmarks, achieving a dissociation between 60 and 80 degrees. They are in the tails of the bell curve. Unfortunately, we lack sufficient insight and evidence at this time to determine whether this increased dissociation offers a significant advantage for increasing pitching velocity. True, preliminary findings by Stodden, Fleisig and others, in a 2001 article, suggest that this increased dissociation could offer some benefit for pitching velocity.[7] For that benefit to be realized, however, the shoulder would need to “catch-up” quickly enough with the rest of the throwing motion to deliver the baseball efficiently.
(4) Stride Length and Leg Lift
Next we consider how a pitcher’s length of stride off the rubber can impact his velocity. Current biomechanical studies show that when pitchers are moving more quickly toward home plate, they tend also to go farther toward home plate, which corresponds to a longer stride length. Ramsey, Crotin, and White, in a 2014 article, argue that it is possible to increase pitching velocity by lengthening one’s stride so long as one also maintains proper pelvis rotational velocity and timing.[16] Of course, there is a point at which a stride length becomes too long, precluding proper, on-time trunk rotation. Conversely, when stride length is too short, a pitcher may have too little linear velocity, likewise precluding proper timing of pelvis, upper trunk, and arm rotations. Biomechanical inefficiency can result from striding too long or too short, and either case leads to diminished pitching velocity.
To maximize linear velocity toward the plate, the pitcher must stride to the right place at right time. These days, most professional pitchers are achieving a stride length of approximately their body height, or six shoe lengths. However, many of the power pitchers today get out even further. Nolan Ryan consistently exceeded seven shoe lengths while putting his cleats in the same spike marks, pitch after pitch. Sandy Koufax is also reported to have exceeded seven shoe lengths. Of course, pitchers don’t simply decide to increase their stride length in isolation from the rest to their pitching motion. Rather, increased stride length is a byproduct of faultlessly executing the other aspects of the pitching motion.
In addition to increased stride length, power pitchers also finish their stride in a distinctive way: power pitchers land with a slightly flexed front leg that then, rather than sagging and acting as a shock absorber, stiffens and rears up. This allows the momentum created up to the point of foot strike to be transferred back through the body into the throwing arm. Baseball insiders call this “recoil” or “bullwhip.” The received wisdom is that recoil or bullwhip is not good for the throwing arm. Who first said it, and where and why, has been lost to time. But power pitchers do recoil.
Evidence suggests that if the follow through remains constant throughout the throwing and release of the baseball, any detrimental effect of recoil/bullwhip on the arm and shoulder can be mitigated or negated completely. The measurements needed to determine recoil/bullwhip focus on the knee angle achieved at foot strike before stiffening the front leg and also on the timing associated with that event, especially with how quickly it occurs. According to Matsuo, Fleisig, and others in a 2001 article, such measurements will yield insights not just into the throwing technique of power pitchers, but also into pitching velocity and pitcher health generally.[8] “Pitchers who recoil are maximumeffort guys,” says Eddie Bane, MLB executive.[13]
Related to stride length is leg lift, namely, how high the pitcher lifts the leg that’s striding toward home plate. Evidence suggests that a higher leg lift can generate more bodily momentum toward home plate and thereby increase pitching velocity (so long as dynamic balance can be maintained). Take the following interaction between pitching coach Tom House and power pitcher Nolan Ryan. Ryan came to House in the late 1980s when both were with the Rangers, claiming that his pitching velocity increased when he raised his stride leg higher. House, doing a computational biomechanical analysis, confirmed Ryan’s claim:
Nolan’s left leg is 19 percent of his total body weight. By lifting it six inches higher, Nolan recruited approximately 3 percent more potential energy into his body’s energy system before starting toward home plate. This translated more energy up through his body when his landing foot hit, giving him more arm speed and power to put on his pitches. How much more? About 2 mph on his fastball. Nolan, as usual, was right. But Nolan’s strength and flexibility are unique. He could keep perfect balance with the higher lift, something most pitchers can’t do. So a high leg lift works for Nolan, but many pitchers couldn’t benefit from the technique because it would adversely affect their dynamic balance.[17]
(5) Arm Speed
When the incremental effects of the previously noted elements are combined, enhanced (and perhaps even greatly enhanced) velocity becomes an entirely realistic expectation. To say that every piece of the pitching motion is moving toward home plate more quickly means simply that the entire kinetic chain is speeding up. It follows that if elements of the kinetic chain prior to the forward arm motion are quickening, then the arm speed of the pitcher is likewise going to quicken, keeping pace with increased speeds earlier in the kinetic chain.
To illustrate what’s at stake here, imagine you stumble while approaching a door. In this case, you have timing, tempo, kinetic sequence, and mechanical movement, with your arm at the end of this sequence coming to your rescue to brace you. But now imagine you attempt to jump over a tennis net but catch your toe on the tape. Again, you have timing, tempo, kinetic sequence, and mechanical movement working for you, but this time the sequence occurs far more quickly, with the arm likewise having to brace you far more quickly (and much more likely to get injured than in the first case).
In both cases, the resulting mechanical movements to protect the body occur on time and without any rehearsal. The same happens with arm speed in pitching. The mind makes the “executive decision” to mechanically move the arm to the right place at the right time depending on prior elements in the pitching motion. The faster those prior elements, the faster what follows.
(6) Release Point
The final area where a pitcher could potentially increase his velocity is the release point of the baseball. Whereas the other five areas discussed earlier could lead to measurable increases in pitching velocity, in fact the release point would not affect a pitch’s measured velocity (which is measured at the point of release), inasmuch as the “heat” or energy on the ball has come from the other five areas leading up to its release.
Nonetheless, if Hephaestus did his work well for Zeus, creating not just the fastest pitcher but the perfect pitcher, then this pitcher is going to “ride” the baseball as long as possible. This means that the fingers, the long fingers, remain on the seams of the ball longer than for the average hurler. Accordingly, while the actual velocity will derive from everything earlier in the pitching motion, the perceived velocity will be greater because the pitcher is releasing the ball closer to home plate. Moreover, by “riding” the baseball longer, the revolution count on the ball would increase. And with the increase of revolutions on the baseball, ball movement would increase and the fastball would appear to rise, leading to a greater proportion of strikeouts, as we would expect from the perfect pitcher.
Conclusion
We have taken great care to build our perfect pitcher, providing him with perfect timing, tempo, and kinetic sequencing. Now what? The authors are persuaded that, given Aroldis Chapman’s 105 mph pitch as our starting point, “finding” an additional 5 miles per hour is plausible and even realistic. Over more than three decades, one of us (BV) has worked with many hundreds of pitchers at all levels, including the MLB, witnessing significant velocity increases when they incorporate improved timing, tempo, kinetic chain, and biomechanical variables. The improvements happen both incrementally, by improving individual pieces in the pitching motion, and aggregately, by coordinating and combining them effectively.
Perhaps Dalkowski was doing things on the mound that we’ve missed or failed to imagine. Perhaps he was sui generis, uniquely unique, a freak of nature. But we think such a conclusion unnecessary. As “Sudden” Sam McDowell noted in the foreword to our book, he didn’t see Dalkowski doing anything unprecedented and inscrutable on the mound; rather, he saw Dalkowski combining familiar pieces of the pitching motion in a remarkably efficient and seamless way. In this and the previous article, we have argued that Dalkowski was not radically different, just radically better at accelerating a baseball. That remains our conclusion.
Addendum: Steve Dalkowski’s Rising Fastball
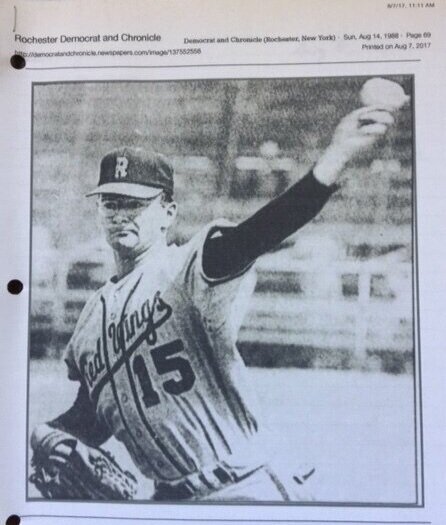
This 1963 photo from the Rochester Democrat and Chronicle was in the stacks of the Dalko authors’ research. This photograph was taken after Dalko had injured his arm in spring training that year. Until the injury, he had been slated for the 1963 Baltimore Orioles Roster. The Orioles sent him down Rochester (his only AAA assignment) to work through the injury.
In this photograph, Dalkowski is releasing a pitch, probably during a bullpen session. Author and pitching coach Brian Vikander warns that “Looking at one frame is ‘quicksand,’ for a pitching coach.” With that caveat, we can notice several things had changed for the better in Dalko’s delivery. Was it his work with Earl Weaver in 1962 or did Dalko simply figure it out?
We will never know for sure but here are some facts we know from looking at this photo:
1) Dalko’s head, eyes, and glove are all going to the target (catchers glove). Most earlier photographs of Dalko show his glove “being soft” and going to the 3rd base side;
2) Dalko’s chest is going to the glove which produces energy and repeatablility;
3) Note the hyperextension in the lower back which also contributes energy and repeatablility;
4) Dalko’s wrist is underneath the baseball. If he could bring his wrist forward with quick energy, this is potentially an element of what he did to generate velocity and create his “rising” fastball. This component is something that teammates and opposing players addressed all the time about Dalko’s delivery, including Cal Ripken, Sr., Andy Etchebarren, and Hall of Famer Pat Gillick.
Endnotes
[1] Fleisig GS, Laughlin WA, Aune KT, Cain EL, Dugas JR, Andrews JR., Differences among fastball, curveball, and change-up pitching biomechanics across various levels of baseball, Sport Biomech. 2016; 15 (2): 128-138.
[2] Diffendaffer AZ, Fleisig GS, Ivey B, Aune KT, Kinematic and kinetic differences between left-and right-handed professional baseball pitchers, Sport Biomech. 2018.
[3] Escamilla RF, Slowik JS, Diffendaffer AZ, Fleisig GS, Differences among overhand, threequarter, and sidearm pitching biomechanics in professional baseball players, J Appl Biomech. 2018; 34 (5): 377-385.
[4] Escamilla RF, Fleisig GS, Groeschner D, Akizuki K., Biomechanical comparisons among fastball, slider, curveball, and changeup pitch types and between balls and strikes in professional baseball pitchers, Am J Sports Med. 2017; 45 (14): 3358-3367.
[5] Oi T, Slowik JS, Diffendaffer AZ, et al. Biomechanical differences between Japanese and American professional baseball pitchers. Orthop J Sport Med. 2019; 7 (2): 2325967119825625.
[6] Urbin MA, Fleisig GS, Abebe A, Andrews JR., Associations between timing in the baseball pitch and shoulder kinetics, elbow kinetics, and ball speed, Am J Sports Med. 2013; 41 (2): 336-342.
[7] Stodden DF, Fleisig GS, Mclean SP, Lyman S, Andrews JR., Relationship of pelvis and upper torso kinematics to pitched baseball velocity, J Appl Biomech. 2001; 17: 164-172. 10
[8] Matsuo T, Escamilla R, Fleisig GS, Barrentine SW, Andrews JR., Comparison of kinematic and temporal parameters between different pitch velocity groups, J Appl Biomech. 2001; 17: 1-13.
[9] Tom House, Gary Heil, and Steve Johnson, The Art and Science of Pitching (Monterey, Calif.: Coach’s Choice, 2006), 29-31.
[10] Tom House and Doug Thorburn, Arm Action, Arm Path, and the Perfect Pitch: Building a Million-Dollar Arm (Monterey, Calif.: Coach’s Choice, 2009), 95-107.
[11] Tom House and Timothy E. Kremchek, Fastball Fitness (Monterey, Calif.: Coach’s Choice, 2007), 26-27.
[12] Tom House, Coaches Certification Clinic August 2011 and January 2012, single volume, published through NPA (The National Pitching Association) and RDRBI (The Rod Dedeaux Research and Baseball Institute), 20.
[13] Paul Dickson, The Dickson Baseball Dictionary, 3rd ed., entry “recoil” (New York: Norton, 2009), 693.
[14] For the average size of contemporary baseball players by position, with contemporary pitchers coming in at 6’ 2.5”, see http://wearefanatics.com/baseball-players-shapes-sizes/.
[15] To see that Jon Rauch’s four-seam fastball averaged in the low 90s, see http://www.brooksbaseball.net/landing.php?player=400010.
[16] Dan Ramsey, Ryan Crotin, and Scott White, “Effect of stride length on overarm throwing delivery: A linear momentum response,” Human Movement Science. 2014; 38: 185-196.
[17] Tom House, The Pitching Edge, 2nd edition (Monterey, Calif.: Coach’s Choice, 2000), 6.